Metal-poor stars and chemodynamical evolution of the Galaxy
(Kobayashi, Yates)
Galactic Phylogenetics
Dr Yates is a member of the ERIS collaboration which studies the chemodynamical evolution of the Milky Way. ERIS is unique in that it uses "phylogenetics" -- a technique originally developed for biology research into the evolution of species -- for Galactic astronomy. Rather than collecting fossils of different species within the same evolutionary tree, ERIS collects stars of different (but largely unknown) ages within the Milky Way and uses their unique chemical composition as a DNA fingerprint. These fingerprints are obtained from spectra taken by spectrographs such as APOGEE and GALAH. Sophisticated galactic chemical evolution (GCE) models then tell us how the chemical composition of the Milky Way should evolve, and thus how to link the stars through a grand "family tree" of our Galaxy.
ERIS has had great success in this endeavour thus far, uncovering a dynamics-free way of distinguishing different components of the Milky Way disc (Walsen et al. 2023), and testing phylogenetic principles on galaxy evolution simulations (de Brito Silva et al. 2023). Future work by ERIS from CAR researchers and collaborators will harness GCE models like that in the L-Galaxies simulation (see Theory and Modelling) to unpick the formation history of the Galaxy and its stellar halo, as well as study the radial distribution of chemical elements in the Galactic disc. ERIS will also extend the phylogenetic technique to other galaxies, by utilising next-generation GCE networks and unresolved stellar spectra from integral-field units such as MaNGA and MUSE. This will set the chemical evolution of the Milky Way in the context of its neighbours better than ever before.
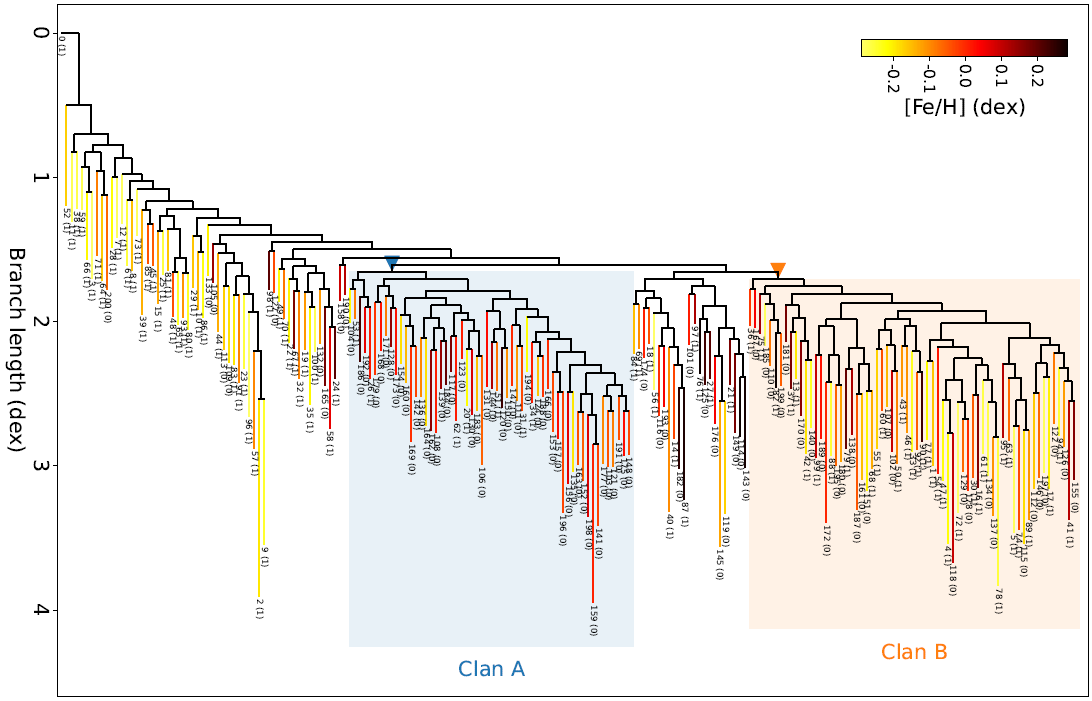
Chemodynamical Simulations
(Kobayashi)
Using chemical evolution models, the time evolution of elements has been well reproduced in the solar neighbourhood with metal-dependent nucleosynthesis yields of (1) core-collapse supernovae for massive stars (Kobayashi et al. 2006), (2) thermonuclear supernovae from low-mass binary stars with the metallicity effect (Kobayashi et al. 1998), and low-mass AGB stars (Kobayashi et al. 2011).
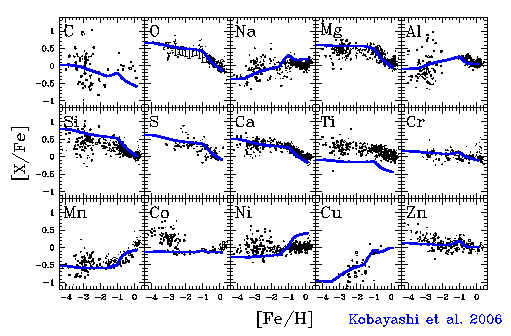
High-resolution multi-object spectrographs such as GAIA-ESO survey, APOGEE, HERMES, WEAVE, in which we are participating, will deliver elemental abundances for millions of stars in the Local Group. In order to understand the origin of the scatter, we need a realistic model.
We study the formation and evolutionary histories of Milky Way-type galaxies using the chemo-dynamical simulations described by Kobayashi and Nakasato (2011). These incorporate Smoothed Particle Hydrodynamics (SPH) to describe hydrodynamics, and direct summation with the GRAPE system or tree method describes gravity. The relevant physical processes such as radiative cooling, star formation, supernovae feedback, and chemical enrichment are all included. This enables these simulations to be compared with observed elemental abundances of stars in various locations - bulge, disk, halo, and satellite galaxies. In the simulations, the bulge have formed by assembly of gas-rich subgalaxies. The disk forms inside-out with a longer timescale. A half of the thick disk stars come from the minor merging of satellite galaxies.
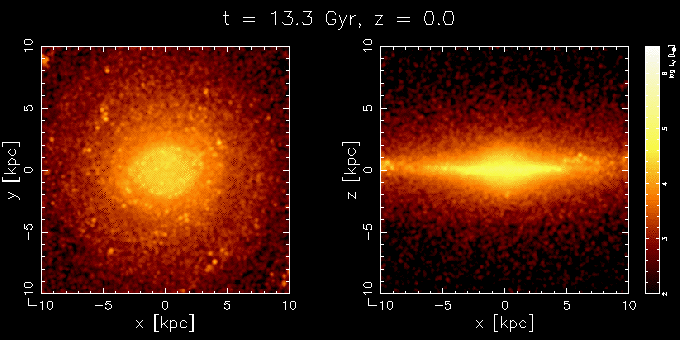
Using our simulations, we have predicted the time/redshift evolution of the spatial distribution of elements from Lithium to Zinc (A=30). This will be extended up to Uranium (A=92) using new AGB yields and r-process calculations (Kobayashi et al. 2015). By comparing with observations, we aim to untangle the star formation and chemical enrichment histories of the Milky Way Galaxy.
This quest is fundamentally tied to the mystery of the origin of the elements, which has a clear connection with the origins of life. Our human body comes 90% from stellar dust. But what kind of stars were they? How did they explode?